Next: Conclusion
Up: Preliminary results of dark-speckle
Previous: Dark speckle lifetime
Table:
Brightness ratio and SNR obtained with a photon-counting avalanche
photodiode, for two values of the sampling
parameter j. The number of zero-photon events was counted on 250000 short
exposures of
, totalling 25s of integration. The SNR was
calculated from Eq.(1).
j |
Rmax |
R |
0 ph. |
0 ph. (star |
SNR |
|
|
|
(star) |
+planet) |
|
|
|
15000 |
136796 |
110377 |
71.4 |
80 |
440000 |
150000 |
87672 |
85546 |
7.2 |
|
|
360000 |
82340 |
80625 |
5.9 |
144 |
790000 |
560000 |
111044 |
107946 |
9.3 |
|
|
950000 |
155959 |
150017 |
4.0 |
To assess the dark-speckle method we did a laboratory simulation using a
single-pixel photon-counting detector, in the form of an avalanche
photodiode.
The star and planet were simulated by two He-Ne lasers, with
adjustable attenuators.
A Lyot-type coronagraph permitted to remove the star's
Airy peak and rings, thus decreasing the local halo intensity 10
to 15 times. Artificial ``seeing'' was generated with a moving
scatterer, selected to provide a Strehl ratio approaching that typical of
current adaptive optical systems. The equivalent peak/halo gain was
G=3.4.103. The flux of the central star was 44.106
photons/s. Calculating an histogram of the detected photon events, we
determined the
SNR by comparing the number of zero-photon events with the planet
turned on and off.
As listed in Table 1, the results strongly depend
on the sampling parameter j. They are consistent with Eq.(7) which gives
the maximum brightness ratio (Rmax) theoretically reachable.
In these laboratory tests, the dark-speckle analysis outperforms the long exposure
when the sampling exceeds 144 pixels/speckle area.
Figure:
Laboratory simulation of stellar companion detection with the dark
speckle method. The artificial companion, 966 times fainter than the star,
is at the center of the dark map, emerging from the halo. A coronagraphic mask,
which hides the star's central peak is visible in the lower right corner. The
blob seen in the upper right part of the field is a ``static speckle''
caused by permanent aberrations and removable with a reference star.
 |
In this experiment the short exposure time (
) is about 100 times shorter
than the speckle lifetime (10ms).
Available photon counting camera do not yet allow quite as short exposure.
Figure:
Cleaned image, generated through dark-speckle analysis, of the
multiple star HD144217(
Sco). A companion (
Sco B) appears
near the masked star image. (mask diameter=0.5'', F/D=1200)
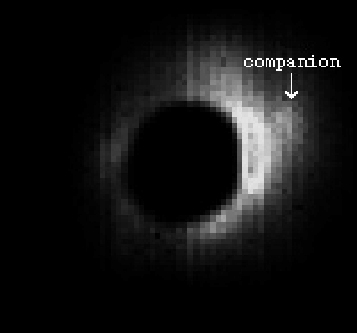 |
We also used the CP40
photon-counting camera developed by Foy and Blazit ([Blazit 1986]). It has pixels
of
and a rather low saturation level of 50000 photons/s. Each
pixel has a lower dark noise than an avalanche photodiode, but a much slower
response. We used the algorithm described in Labeyrie (1995),
generating a ``dark map'' by counting, in each pixel, the number of 20ms
exposures which contribute
zero photon. As contributions from
successive 20ms exposures are accumulated in the dark map, a planet's
Airy peak is expected to emerge as a black dot among background
noise.
To obtain the cleaned image, results are displayed in positive form
using, for example, an inverse square law.
The CP40 discriminates between events featuring zero photoelectron and those
featuring one or more photoelectron/pixel/exposure. Adding all exposures generates
an image which brings out faint companions better than would a similarly
long exposure on a CC
D detector.
The flux of the central star was
5.3.106 photons/s, and the gain of the adaptive optics was about
1556. The optical system operated at
, F/D=3200, and the mask diameter was 0.34", i.e. it
covered the central 2 rings of the Airy pattern.
The planet was located near the fifth ring of the diffraction pattern.
Figure 2 shows that a companion 966 times fainter is well detected in
116 seconds.
The SNR measured on a speckle size region (
pixels) is 799,
while the
dark-speckle model predict an SNR of 1008. This model does not take into
account the halo shape which can explain the
discrepancy.
These initial results, where the companion is brighter than the halo, are very
modest with respect to the performance expected at a later stage, but have
provided useful insight for improving the instrument.
Currently, as seen in Figs 2 and 3, the detection sensitivity is limited by the presence
of spurious blobs in the cleaned image. These are caused by static aberrations
and coronagraphic mask effects. These residual blobs may be substracted from
data obtained on a reference star.
Finally, dark-speckle data have been recorded at the 152cm telescope
of Haute-Provence, using, during a single night, the BOA
adaptive optics system ([Madec 1997]) developped by the Office National d'Etudes
et de Recherches Aérospatiales (ONERA).
Figure:
Same image enhanced with unsharp mask and high pass filter to emphasize
the contrast and median filter to smooth the image at the speckle scale.
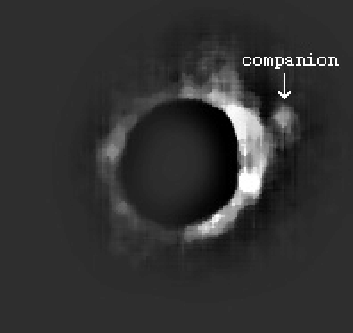 |
This system, optimized for visible light, reaches a Strehl ratio of
about 0.4 at
in long exposures and higher in short exposures. 30
minutes of observation, with an interference
filter centered at
(
), evidenced the
faint component of the spectroscopic binary HD144217 (
,
, V=2.62). On the detector, the flux from the
primary was only 11860 photons/s.
The angular separation is about 0.45" with an uncertainty due to the
mask offset. Owing to the low elevation of the star, the adaptive optics gain
was only 12. The SNR measured on
pixels is 168 and allows
to derive
from Eq.(10) a brightness ratio of 88, corresponding to a 4.8 magnitude
difference. On the long exposure synthesized from the same data
(1 photon-events analysis) the SNR is very similar, but a direct imaging
should give a SNR of 42 according to Eq.(11).
However, a recent measurement of lunar-occultation ([Evans 1983]) gives
. In this case, dark-speckle analysis should provide a very good detection
with a SNR of 756 instead of 168.
Unfortunately, the Hipparcos mission failed to detect the companion, probably
because the Hipparcos satellite is unable to achieve
with small
angular separation, which is consistent with our data.
More observations are needed to verify the companion magnitude.
A continuing observing program is initiated.
Next: Conclusion
Up: Preliminary results of dark-speckle
Previous: Dark speckle lifetime
6/10/1998